Nerve Cells Show Deficits at Early Disease Stages, Mouse Study Finds
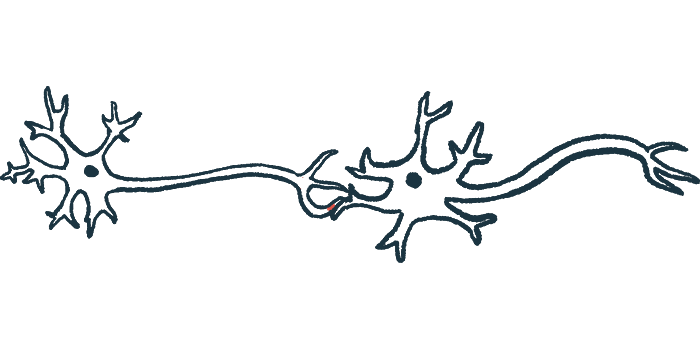
Nerve cells in brain tissue from a Sanfilippo syndrome type C mouse model — in early stages of the disease — found structural abnormalities and deficits in nerve cell signaling.
These impairments, the study’s scientists said, might underlie the early learning and behavior symptoms seen in Sanfilippo patients.
The study, “Early defects in mucopolysaccharidosis type IIIC disrupt excitatory synaptic transmission,” was published in the journal JCI Insight.
Sanfilippo syndrome, also known as mucopolysaccharidosis type III (MPS III), is caused by mutations in one of four genes that encode enzymes that break down the complex sugar molecule heparan sulfate.
Because of these mutations, heparan sulfate or its metabolites build to toxic levels inside lysosomes, which are specialized recycling compartments within cells, and damage nerve cells (neurons) in the brain. As a result, patients experience the hallmark cognitive decline that appears in later disease stages.
It is thought that early impairment in neurons also underlies some initial disease symptoms, including learning difficulties and problematic behaviors. Investigating the earliest changes in nerve cells affected by heparan sulfate buildup may lead to ways to treat these symptoms or prevent the loss of neurons altogether.
Researchers at the University of Montréal in Québec conducted a detailed analysis of neurons isolated from mice with mutations in the Hgsnat gene, the underlying cause of Sanfilippo syndrome type C in people.
This study was supported by funding from the Canadian Institutes of Health Research, Sanfilippo Children’s Research Foundation (Canada), JLK Sanfilippo Research Foundation (U.S.), and Jonah’s Just Begun (U.S.).
Sanfilippo type C animals develop hyperactivity and learning difficulties between 5–6 months of age, followed by urinary retention and death at 10 to 11 months. Notably, the loss of neurons in these mice only becomes significant after age 10 months, whereas behavioral abnormalities are seen as early as 4 months.
Synapses of neurons isolated from the hippocampus, a brain region important in learning and memory, were examined initially. Brain cells communicate through synapses, contact points between neurons where signals are passed from one neuron to the next.
The first neuron sends signals from a synaptic terminal, which is found at the end of a nerve fiber, or axon, that extends from the neuron. The second neuron receives the signal via a dendritic spine, a branched protrusion on the nerve cell body.
Cultured neurons from Sanfilippo type C mouse brains showed the characteristic buildup of heparan sulfate and metabolites within lysosomes, which was not present in cells from healthy control mice.
Dendritic spines were measured 10 and 20 days after birth, as well as at ages 3 and 8 months. As early as 10 days, the density of dendritic spines in Sanfilippo mice neurons was reduced by about 22% compared to controls. This difference increased to 56% at 8 months. The majority of dendritic spines in mutant mice were found to be immature.
Similar changes in dendritic spine density were seen in neurons isolated from mouse models of disorders caused by the buildup of other metabolites in lysosomes, such as Tay-Sachs and sialidosis. “These cells do not accumulate [heparan sulfate], suggesting that spines are not reduced exclusively due to storage of this metabolite,” the researchers wrote.
There was also an abnormal distribution and density of signaling proteins in Sanfilippo type C mice neurons, typically found in the synaptic terminals.
Next, the density of synaptic vesicles — packages of molecules (neurotransmitters) that transport signals across the synapses — at the synaptic terminals of hippocampal neurons were measured in 3- and 6-month-old mice.
Their density was decreased by 22% in 3-month-old Sanfilippo mice compared with controls. This reduction was even more prominent at 6 months, reaching a 30% difference.
Researchers also found alterations in the distribution of excitatory synaptic markers, which are proteins involved in the activation of receiving neurons. In contrast, there were no changes in inhibitory synaptic markers associated with the suppression of receiving neuronal activity.
Excitatory and inhibitory signals are responsible for maintaining adequate stimulation of brain nerve cells. Excitatory signaling from one nerve cell to the next makes the latter cell more likely to fire an electrical signal, while inhibitory signaling makes the latter cell less likely to fire. This is the basis of nerve cell communication in the brain.
To confirm its relevance, an excitatory synaptic marker was also found to be significantly reduced in post-mortem brain samples from Sanfilippo patients.
Additional experiments analyzed the electrical signaling capacity of brain cells from Sanfilippo type C mouse brains. Relative to controls, the strength of their electrical signals was reduced by 32% between 14 and 20 days after birth, and by 43% up to 60 days. Likewise, the frequency of signals significantly decreased at both these time points.
An analysis into the molecular mechanisms underlying synaptic deficits in these mice found major reductions in proteins associated with energy-producing mitochondria (cells’ powerhouses), synaptic proteins, and proteins involved in the trafficking of synaptic vesicles at 3 and 6 months old. Defects in microtubules that support cell structure were also seen. They were disorganized, sparse, and nonparallel.
Finally, to establish a relationship between the deficits in the production of protein markers for excitatory synapses and HGSNAT deficiency, the team attempted to fix these impairments by gene therapy. Using a virus that overexpressed (over-produced) the normal human HGSNAT enzyme, the deficits in excitatory synaptic markers were rescued in Sanfilippo type C neurons, both in cell cultures and in animals.
“Together, our results demonstrate that lysosomal storage defects cause early alterations in synaptic structure and abnormalities in neurotransmission,” the researchers wrote.
“In light of these findings, it is tempting to speculate that drugs known to enhance excitatory synaptic transmission should be tested for their ability to improve synaptic function, as well as behavioral and cognitive defects, in MPSIIIC mice and in other animal models of neurological [lysosomal storage disease].”
If proven effective, “such synaptic targeted treatment — although it would not eliminate the lysosomal storage and substitute for gene correction — may, nevertheless, improve behavioral deficits in patients with advanced form of the disease,” they added.